
We all live the beautiful dream of a hydrogen economy, but it can very easily turn into a nightmare.
The analysis below, which is one strictly from an energy point of view, is based on well-known laws of physics and thermodynamics, easily verified and simplistically displayed for easy and good understanding even by non-specialists, and reveals the huge (physical) barriers that stand in our way along with other huge economic barriers. Technically, anything is possible, and if it’s free money, “who cares to lose it”?
- With a H2 density of 0.0887 kg/m3, it turns out that the classic 11.1 m3 tank that we see on the roads every day can under normal conditions carry an extremely unbearable weight 1 kg of H2!
- If we want to transport in pipelines, i.e. flow rate in m3/h (as methane CH4), then H2, which has only 3.53 kWh/m3 compared to 11.11 kWh/m3 for methane, gives energy intensive through the same gas pipeline at the same flow rate, I only deliver about 31% of the energy we used to deliver with CH4!
Let’s move on to other aspects, leaving aside these “non-essential” details.
- PRODUCTION OF GREEN HYDROGEN
Let’s start the analysis of green hydrogen production with: electrolysis of water (pure!) with electricity (pure!)
The standard voltage for electrolysis is U=1.23 V, and for operational operation a voltage of about U=1.76 V is required for 1 unit of CSP H2, as a result of which 1.43 units of energy must be provided for the production of 1 unit from PCS hydrogen. , that is, the output of the process is 1/1.43= 70%. So:
Electrolysis yield: 70% representing the ratio (H2 energy produced / electrical energy consumed)
NOTE: They are not included losses from high-voltage conversion of alternating current into direct current, necessary for electrolyzers
2 “PACKAGING”
a. Compression (only let’s not transport 1 kg of H2 with a tank of 11.1 m3)
Compression of any gas requires energy consumption, and if we read Table 1 again, we will see that for the adiabatic compression of diatomic hydrogen (V0=11.11 m3/kg) and pentaatomic methane (V0=1.39 m3) / Kg), objectively, it takes much more energy to compress H2
Data provided by compressor manufacturers indicate that a 5-stage compressor with a hydrogen flow rate of 1000 kg/h increases the ambient pressure to 20 MPa (1 megapascal = 10 bar) using electricity equivalent to 7 .2% PCS H2. Adiabatic and isothermal compression (both must be considered) essentially lead to total loss 8%
Compression energy consumption – adiabatic/storage isotherm = 8% hydrogen PCS. value
At least 1.08 units of energy are required to produce 1 unit of H2 at PCS at 20 MPa.
Note: the analysis does not include the resulting electrical losses from the conversion of power supply from the electrical network of the corresponding compressor
If the 80 MPa standard required to meet the 70 MPa automotive industry tank standard is adopted, then 1 unit of H2 at PCS at 80 MPa will require 1.12 units of energy.
As numbers to visualize the process and scale of the problem, in Texas (USA) Air Products and AES have built a plant to produce H2 and compress 200 t H2/day (i.e. 8333 kg H2/h, i.e. 333.3 MW). ), which is powered by a wind and solar power plant with a capacity of 1400 MW.
That is: 1 MW of green hydrogen requires at least 4.2-4.5 MW of renewable energy.
or:
At a 45 t/d H2 plant powered by hydroelectric power, they are minimally needed. The electrolyzer with a capacity of 120 MW is constantly fed by the hydroelectric power station
That is: for the production of 1 MW of green hydrogen, a minimum of 1.6-1.7 MW of hydropower plants is required.
or:
A plant with a lower capacity, 4.4 t/day of hydrogen (ie 182 kg/h = 7.3 MW), requires 2.73 MW (green!)
That is, for the production of 1 MW of green hydrogen, a minimum of 2.7 MW is required
An interesting aspect is that at low yields (about more than 5 kg/hr of liquefied H2), the energy required for liquefaction exceeds the net energy obtained with hydrogen!
b. Liquefaction
In this case, the process requires even more energy, in a complex and expensive process.
Liquefaction (by cooling) of H2 is achieved with the help of multi-stage compressors and expansion (expanders) in combination with countercurrent heat collectors and energy recovery (through expanders – expansion turbines by reducing pressure). As an example, a Japanese feasibility study showed that for a quantity of 12,500 kg/h of liquefied H2 (approximately 500 MW equivalents), we need a power plant of about 105 MW (green!)
Only for operation of H2 liquefaction capacities!
in. Physical metal hydrides
We will not study this method of storage here, as it is extremely complex and expensive
3 distribution
a.Automobile transport
The economy based on H2 should include road, rail, sea transport. Absolutely necessary, road transport is an objective necessity.
A modern 40 metric ton tubular tank transports 3,000 kg of methane at 20 MPa pressure, delivering only 2,400 kg (80%) to the customer as the tank is emptied until the pressure in the tank and tank reaches the customer. levels!
The same tank can only transport 320 kg of hydrogen at a pressure of 20 MPa, but will actually deliver 288 kg of hydrogen. Therefore, however, compressors will be required to completely empty the tank, which involves other energy consumption (other equipment, procedures and additional consumption)
As a preliminary conclusion: while road transport is generally limited/dictated by weight, in the case of hydrogen the paradigm is different: the limitation is set by volume, not weight, due to the extremely low specific volume of hydrogen
Today, approximately 1 tanker out of 100 carries gasoline or diesel fuel
For the transportation of hydrogen, 15 equivalent tankers will be needed, that is, 115, which means an increase in traffic by 13%. Hence, 1 in 7 tank accidents may involve hydrogen tanks and 1 in 49 accidents may occur between 2 hydrogen tanks, a scenario and probability calculation that is totally unacceptable for many reasons! (heavy traffic, bridges, tunnels, traffic through specific terrain for Romania, lack of highways, etc.)
b. Pipeline transport
Hydrogen pipelines already exist today, but they are designed to be transported between 2 production/consumption locations over relatively short distances, and the energy consumption is irrelevant as it is part of the production process of the facility in question.
But this is not at all the same as transporting hydrogen from one business entity to another over any distance!
As a rule, the transportation of natural gas (CH4)-methane through a pipeline at a speed of 10 m/s requires a compression station every 100-200 km, which consumes approx. 0.3% of transported energy (for example, the famous BRUA pipeline, which would transport 4 billion m3/year of natural gas from the Black Sea to Hungary, would consume only 1 of 3 compressor stations powered by 5 MW gas turbines, about 0.3% x 4 billion m3 /year = 12 million m3 of gas/year/compression station (so almost 1% of transit energy – a big number!) is used for compression/transportation). What is interesting is that as a result CO2 emissions approx. 9 million euros/year, not taken into account for their payment by Transgaz !?)
Applying the rules of physics, for the same hydrogen transport section, each compression station would require 1.16% of the consumption for compression/transportation (ie about 3.4% of the energy transported)
Or, as a conclusion, for a 3000 km long pipeline, the mass fraction spent on methane transportation is approx. 20%, while for hydrogen it is approx. 34% (result for the same pipe diameter).
Or the energy used to transport 1 PCS of hydrogen unit over a distance of 3000 km, at least 1.5 units of energy must be invested to deliver 1 PCS of hydrogen unit.
Since energy consumption for long-distance hydrogen is very energy-intensive, optimizing the length of the routes must be left to engineers and experts. Also, hydrogen leaks are much higher due to the very low specific gravity, and if the seals are not perfect, this factor alone can economically and energetically destroy any longer transport pipeline project! We do NOT discuss other technical and physical problems associated with transporting H2 through pipelines.
4 storage
Analyzing this option is probably the easiest and most obvious comparison.
Based on the above, it was stated that, in general, the efficiency of obtaining hydrogen, its transportation and storage is approximately 50%, before its preparation and use in an electricity production plant (a CHP with a net yield of 50%) will reach a net result of a maximum of 25% .
It is worth noting that even in Romania there were ideas of storing H2 in caverns (former salt mines). We don’t know exactly what this “idea” refers to, but turning salt mines (like Slanik, or Pride, etc.) into real health and healing locations into hydrogen tanks is an awkward idea, without further ado, consider the technical and physical problems of the real storage in such “places”
Pumped storage hydroelectric storage currently has an 80% cycle efficiency for storage and conversion directly to electricity, so it can’t even be compared to H2 storage! –
Read the whole article and comment on Contributors.ro
Source: Hot News
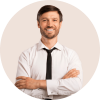
James Springer is a renowned author and opinion writer, known for his bold and thought-provoking articles on a wide range of topics. He currently works as a writer at 247 news reel, where he uses his unique voice and sharp wit to offer fresh perspectives on current events. His articles are widely read and shared and has earned him a reputation as a talented and insightful writer.